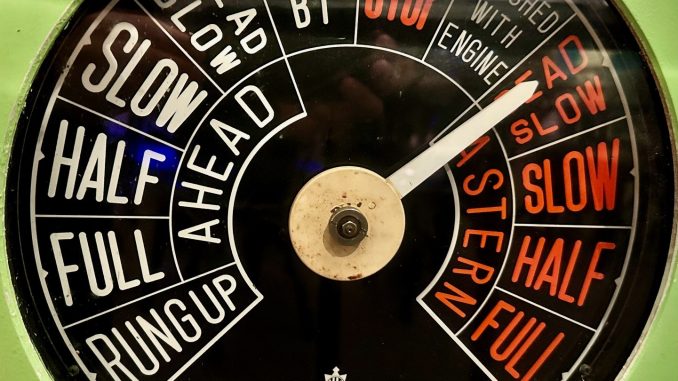
This year, I’ve become part of a peer review panel. These are panels that wade through a long list of proposals for beamlines, and rank the proposals. Based on this ranking combined with the amount of available beamtime, proposals get accepted or rejected. My involvement gives me some insight into how others design their experiments, and… I find myself having opinions on issues that exist across many proposals that I thought might be worth addressing.
[beamline managers; if you want to use this or adapt this as a handout, please feel free but include a link to the lookingatnothing.com blog, thanks! – BRP]
The main criteria
There are a few criteria that go into these rankings. In brief, the following items are some of the more important items that are checked by the peer review panel:
- Need for synchrotron radiation,
- Societal, industrial, or scientific relevance,
- Consideration of the experimental and data analysis plan
Contrary to public opinion, panels don’t evaluate based on length or text density of the proposal. In fact, if you manage to convey your information in a concise, short manner, you make the panel’s life easier. Other short tips include: 1) add some proof-of-principle data, and 2) definitely, absolutely talk to a beamline staff member about your experiment and listen to their opinions.
Now, let’s discuss the main items in turn, and I’ll add my anonymized experiences.
Do you really need a synchrotron?
The main advantage of a synchrotron is the enormous flux, small beams, and the tunable energy (discretized for undulator beamlines). If you are studying fast, dynamic phenomena on the second time-scale or less, you need a synchrotron. If you want to do extensive mapping experiments, you need a synchrotron. The last issue is a bit more complex.
If you have samples with fluorescing elements (metal ions, etc.), check their excitation and fluorescence energies. The direct detection detectors common in beamlines now can filter out photon energies, but you need a few keV for that. So if you have fluorescing iron, you might need a syncrotron so you can set the photon energy to around 18 keV, and use the default detector filter energy at 9 keV to suppress the iron fluorescence at 7. Similar considerations can be found in my video explainer here.
If none of the above applies to your experiment, a laboratory instrument might well suffice and will give you data much faster than the whole proposal-deadline-review-acceptance-scheduling-experiment timeline would. Try finding an amenable, proximate laboratory instrument and discuss with the instrument responsibles to get their recommendations and/or insights. The MOUSE, for example, can churn through about 700 static samples per year, and can also study slow kinetics and do some in-situ experiments. This or similar equipment may be accessible to you too.
What is the societal, industrial or scientific relevance?
Synchrotrons are energy-hungry beasts (some requiring the same power as a small village). We must use this power with great responsibility, and make the results worth the environmental impact of the experiment. This relevance should be clear from the proposal.
To clarify a little, societal relevance could be working on (improving of) materials such as those used for water filtration, energy storage, or energy generation materials. Industrially relevant materials essentially is anything which is in use in, or (close to) being manufactured by industry. Scientifically relevant materials are those that can help to answer a fundamental scientific question.
Conversely, if you are doing your experiment because 1) your direct supervisor told you to, which happens more often than you think, or 2) you have a working method and want to crank out a new paper with a slight change, you might want to reconsider. Changing chemistry for a known phenomenon is not inherently bad, provided you thereby open up a new dimension of knowledge. For example, choosing chemistries that now span a range of polarities because you expect different behaviour, that might be interesting indeed. Random shots in the dark? Not so much.
What is your plan?
Having justified your need for a synchrotron and the relevance of your experiment, we now need to know that you’ve put some effort into thinking about practical aspects of your synchrotron experiment.
These include details on how much time you plan on setting up, time per sample, do you have some spare samples just in case it goes smoother than expected, did you prioritize your samples etc.. Also very important: did you talk to a beamline staff member about your experiment idea, its needs and your plan? Did you listen to their responses and integrated their concerns and ideas into your plan?
Don’t stop at data collection
As for me, personally, the experiment does not start or end on the synchrotron. You need a solid plan of what to do with the data you get. If you are doing mapping (or worse, tomography) experiments, can you even handle the data? For normal synchrotron experiments such as in-situ experiments, it really helps if you have preliminary data, and if you have a model that demonstrably can describe that. Show the preliminary data, show the model, and you get some bonus points in the evaluation for sure.
Avoid reactions in capillaries
A second experimental issue that I stumble across regularly in these proposals is that many propose doing experiments in capillaries. This has two issues.
Firstly, these reactions, being so much smaller in reaction volume than normal laboratory-scale reactions, are often not comparable. When I was studying chemical engineering, up- and downscaling of chemical reactions is explained as a black art: due to changes in the reactors, mixing, wall interactions and so on, reactions do not compare at different scales. This problem is compounded by the capillary heating not being well-controlled and very localized (think hot air blowers at around the capillary center with a large gradient across). These issues can easily be alleviated, in the same way as problem number two:
The second problem is that of beam-induced effects (see this paper, for a summary of these effects). These effects are highly likely with the exceedingly bright, focused beams now available even at your garden-mill variety synchrotron. This beam will change the kinetics of the reaction and of the initiation of processes such as crystallization, and so, even if you get nice results, there is no guarantee they are comparable with such reactions in the outside world.
These two aspects can be drastically reduced if you do your reaction on a more relevant laboratory scale on the side, say at 10 ml or 20 ml, and then pumping this continuously through the measurement cell. This solution isn’t universally applicable, as it may suffer from blockage if you precipitate a lot of material, or if you have strongly gelling samples. However, for many of the experiments I see proposed, it will work just fine and would give you much more valuable, relevant data.
Focusing beam or low q, but not both..
Some beamlines boast a low minimum scattering vector Q value, and also offer focusing options on their beam for a small spot size on the sample. These are not possible at the same time, though.
If you focus your beam, you introduce divergence to the beam. the spot size will be nice and small at the sample position for your mapping experiments but will expand beyond that point. With long-focusing optics, the divergence will not be that bad, but your resolution will suffer, and you will lose your lowest-Q resolution. So choose wisely.
Don’t ask for more time than you need
This again falls down to the experimental plan, but if the panel gets the impression you’re asking for more time than you need, the panel will recommend shortening your time. And do you really want people estimate how much time you really need, who’ve only barely begun to understand your project?
So make sure the samples you bring are the samples you need to address your scientific question, and that the time you ask for is a reasonable estimate for measuring those samples. Don’t come with hundreds of samples when only a few will do, don’t ask 30 minutes per sample if you can measure them on a rack in a minute. Also don’t come with multiple similar proposals if only one will do. Your chances don’t increase, and your experimental plan might be put to question.
Designing sample series
There is a trick to designing sample series. I often see people proposing samples with four different values of parameter X (e.g. synthesis time), and then bringing three repeats of each sample to get some idea about experimental uncertainty. These 12 samples are pointless for two reasons:
One: for anything but the most basic, linear relationship, four samples is far too few to see a trend. If there is any deviation from linearity, your experiment is toast.
Two, and very similar: three datapoints are far too few to get any idea about the experimental uncertainty of your processes. If you try and make error bars from three repeats in total, your error bars will suck, and it will seem to me that you only made them for show rather than merit.
What you can do, however, is to prepare 12 different samples in that series. That way, if there is a linear relationship, you can fit it, and get an idea about the uncertainty from statistics on all 12. If there is a more complex relationship, you will see it, and still get a better idea about uncertainty than with the previous proposal.
On the other hand, you could just robotically prepare 1200 samples and get all the statistics you could ever want!
In summary
It is not hard to make a successful synchrotron proposal, as long as you take care of those three items: need, relevance, and planning. Your friendly neighbourhood beamline manager will also be very happy to discuss these aspects before your proposal with you, no matter if you are a beginner or an expert. Do not add elements to your proposal that might piss off the panel, and you’ll be just fine.